Computational insights into mixing: How process engineers are leveraging simulation in multiphase systems
Mixing in a multiphase system, especially in a scale-up environment, can prove to be an uphill task. A thorough understanding of mixing patterns is crucial as it enables the optimization of process parameters, reactor design, and operational conditions. It also aids in controlling phase separations and preventing undesirable side reactions. Process engineers are leveraging simulation tools in multiphase systems to fine-tune essential parameters to achieve the first-time right scale-up and enhance process efficiency.
Solid-Liquid-Liquid Systems: A Complex Phenomenon
A solid-liquid-liquid multiphase system refers to a complex arrangement where three phases—solid, liquid, and liquid—coexist. These systems are intriguing due to their unique properties and significant impact on reaction conversions. While solid-liquid or liquid-liquid systems are more common, solid-liquid-liquid systems are relatively rare occurrences. The solid-liquid-liquid systems offer enhanced mass transfer and interfacial area, facilitating efficient chemical reactions. These systems find applications in pharmaceutical manufacturing and an understanding of the mixing dynamics in solid-liquid-liquid multiphase systems is of great significance.
Importance of Efficient Mixing
Efficient mixing plays a crucial role in promoting mass transfer and ensuring uniform distribution of reactants, thus enhancing reaction rates and conversions. It helps overcome diffusion limitations and facilitates contact between the solid particles and the reactants, leading to improved selectivity and overall process efficiency.
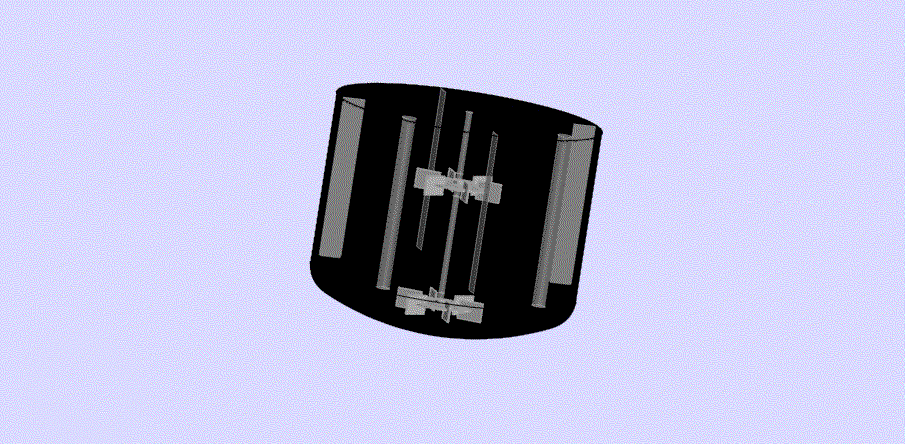
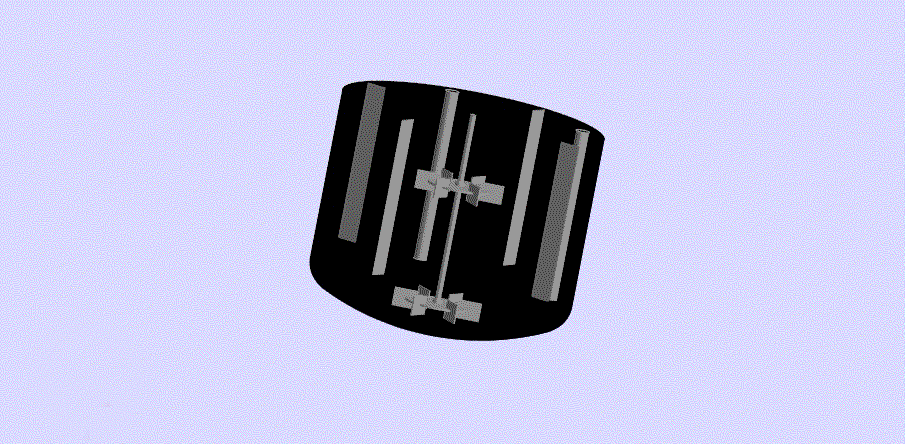
Source: MixiT
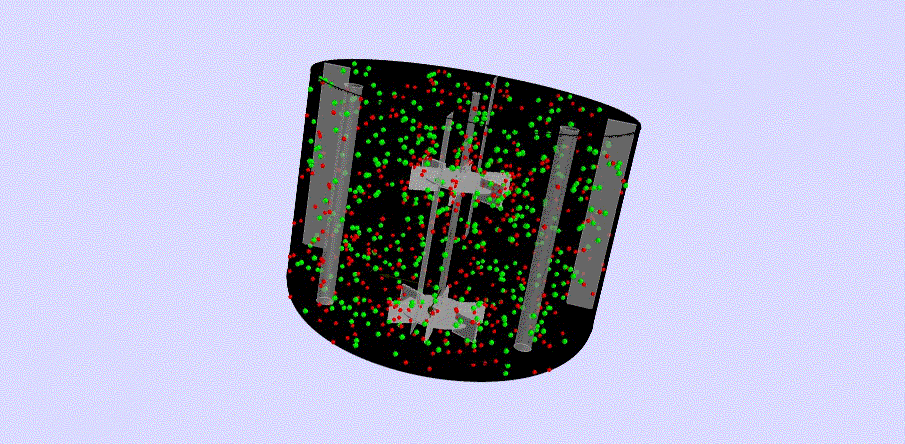
The ‘Scale-Up’ Challenges
In general, at the lab scale, physical observation gives a fair idea in deciding the speed of the impeller and the possibility of adjusting the speed is easy. Whereas, in a scale-up, selecting a suitable type of impeller and determining the speed of the impeller for good mixing in the plant reactor is challenging. Hence, an understanding of the minimum speed of the impeller to lift the solids to hold them in a suspension state (just suspension speed (Njs)) and the minimum speed of the impeller to disperse the less dense liquid phase into the high-dense liquid phase adequately (just dispersion speed (Njd)) is crucial for effective mass transfer as well as reaction conversion.
Computational Fluid Dynamics Simulation holds the Key
The Computational Fluid Dynamics (CFD) simulations conducted using MixIT software facilitate a comprehensive analysis of the plant reactor's mixing process.
By simulating diverse operational scenarios, engineers gain insights into the impact of impeller design, impeller speed and baffling configuration on flow behaviour and mixing efficiency. The CFD outputs provide visualization of flow patterns, allowing one to identify the homogeneous distribution of solids and uniform dispersion of the liquid phase throughout the reactor. This detailed understanding empowers us to optimize the reactor's geometry and operational parameters, leading to improved reaction kinetics and enhanced product quality. The utilization of CFD-based virtual modelling and simulation has proved instrumental in reducing costs, minimizing process development time, and ensuring efficient scale-up of the process.
Various Tools We Deploy
Sai Life Sciences utilizes a range of software tools such as MixiT, Dynochem and AspenOne to anticipate process performance under varying scales and operating conditions.
MixiT, a CFD-based simulation software, is used to optimize mixing operations in various multiphase systems by predicting critical parameters such as mixing time, power consumption, and the efficiency of mixing systems, along with velocity and flow distribution contours. Based on the output obtained from the MixiT simulation, engineers select the appropriate mixing equipment, optimize process conditions, and avoid mixing issues during scale-up. In particular, while using MixIT, engineers analyze and optimize fluid flow and heat transfer in process equipment. This enables the engineers to evaluate the effects of scale-up on fluid dynamics, identify potential obstacles, and optimize equipment selection and process conditions, leading to improved process performance.
Dynochem finds extensive application in analyzing and optimizing chemical reactions, crystallization, drying, and other unit operations. The software enables engineers to predict process performance by considering factors such as heat transfer, mass transfer, and reaction kinetics. Engineers leverage Dynochem to determine optimal process conditions, assess scale-up feasibility, troubleshoot production issues, and ensure the smooth operation of critical processes.
Aspen Plus plays a vital role in process simulation and modelling during the process development to understand the thermophysical properties of the new molecules. The software is also used to simulate the processes such as distillation, extractions and design the equipment to improve the process efficiency.
Conclusion
Through process simulation, engineers can fine-tune essential parameters to achieve the first-time right scale-up and enhance process efficiency. These tools are used during the process development to develop a robust scalable process, facilitate tech transfer, and ensure smooth execution at a higher scale.